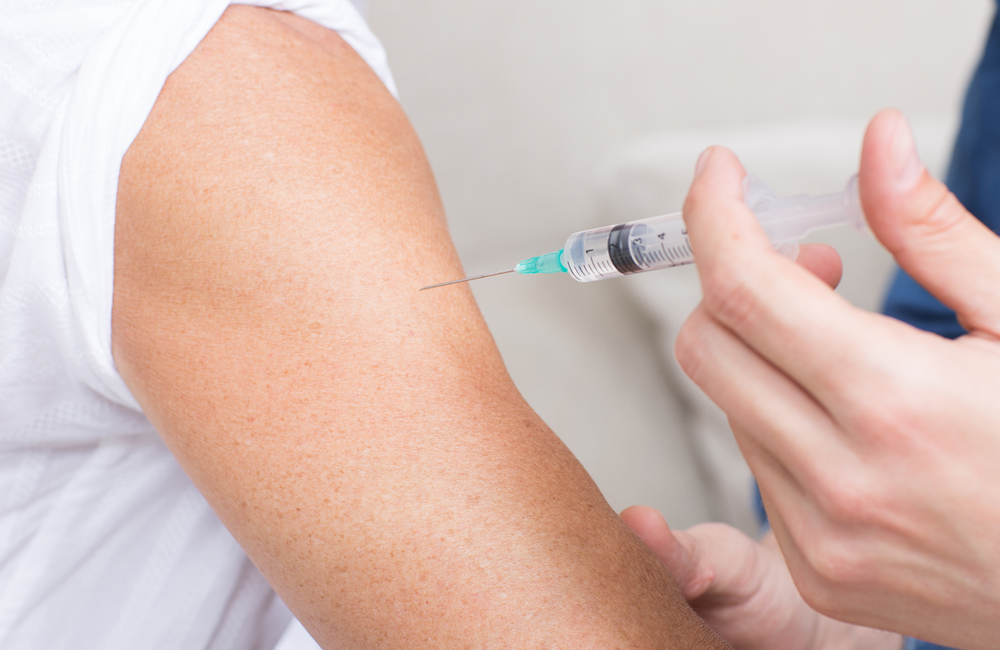
Key points
- Developing a preventive vaccine against HIV is a scientific challenge owing to the virus’s unique properties.
- Most researchers view it as a realistic long-term prospect, though we cannot predict when an effective vaccine will be developed.
- The first vaccines may only protect a proportion of people, or work only against some viral strains, and the protection they confer may need to be boosted regularly. Over time, vaccine efficacy may improve.
- A successful vaccine will need to be active against a wide variety of viral strains. It will probably need to stimulate several branches of the immune system to respond to HIV.
- Two large trials of candidate vaccines closed in February 2020 and August 2021 because of lack of efficacy. A third large trial is ongoing.
- If the third trial’s results are disappointing, this suggests that scientists will need to focus on vaccines based on different strategies. They are being developed, but large trials are unlikely to start for several years.
A vaccine that prevents HIV infection is not available yet. Researchers remain confident that a vaccine that will protect people from infection is possible, but it could be years or even decades away yet.
This page summarises the current state of research and the challenges facing scientists.
It has been difficult to develop a vaccine against HIV for several reasons. Whereas most other vaccines work by teaching part of the immune system to produce antibodies that clear an infection, antibodies are unable to clear HIV infection – indeed their presence indicates infection. This is because HIV generally mutates too rapidly for antibodies and other immune responses to contain infection in time.
Vaccine approaches that have been successful against other viruses – killed or weakened versions of a virus – have not proved suitable in the case of HIV, owing to the risk that the viral material used in the vaccine will integrate into human cells and eventually lead to new virus replication.
Another challenge is that HIV is divided into families, or subtypes, that predominate in different parts of the world. A vaccine will need to be effective against all subtypes, or different vaccines must be developed against different subtypes. Even in one geographical area, a vaccine that only protects against some viral subtypes may not work against others also in circulation. This is not a problem unique to HIV: it is the reason, for instance, why a flu vaccine needs to be adapted each year for the strain thought most likely to be circulating the following winter.
To be effective, an HIV vaccine may need to stimulate all three branches of the immune system to work together:
- Innate immune responses, the fastest and least specific ones, react to harmful invaders by secreting defensive chemicals, stimulating the production of nonspecific antibodies, and mobilising natural killer (NK) cells to destroy cells that bear signs of infection.
- Antibody responses, which are attuned to the specific proteins that viruses or bacteria display on their surfaces. Although the typical antibody response to HIV is usually too weak, too narrow and arrives too late to prevent infection, so-called broadly neutralising antibodies, that recognise parts of HIV that do not mutate, develop in time in some people. If these were produced immediately upon exposure to HIV, they might prevent or contain infection. One goal of HIV vaccine research is to induce the body to make them immediately.
- Cellular immune responses, consisting of CD4 T-lymphocytes that recognise HIV and stimulate other T-cells, such as CD8 cells, to destroy virus-infected cells. Cellular immunity may be the most persistent form of immunity but may work more slowly. It does not actually prevent infections, but reduces the damage they cause and can eventually eliminate them.
We know an effective immune response to HIV is possible because it exists in so-called ‘elite controllers,’ people who live with HIV for many years without any immune damage and with viral loads below 200, without having to take antiretroviral therapy. There may be several different mechanisms of elite control, but most elite controllers seem to have strong virus-specific T cell-mediated immune responses.
A T-cell-stimulating vaccine could lead to the efficient destruction of any cells that HIV antibodies had failed to protect, or it could lead to lower levels of HIV in people who became infected despite vaccination, effectively turning them into elite controllers.
What has been learnt so far about how to produce an effective HIV vaccine?
Vaccine researchers have learnt much from animal studies, from investigations of people who have been exposed to HIV without becoming infected, from HIV controllers, and from clinical trials of experimental HIV vaccines.
Numerous approaches to vaccine design have been tested to learn more about how to protect against HIV and how to produce strong immune responses against HIV. Vaccine trials have investigated the following questions:
- How can a vaccine introduce HIV genes or proteins into the body safely?
- How many doses of vaccine are needed to make a strong immune response?
- Might a combination of vaccines, given in a specific sequence, produce a stronger response?
- What combination of HIV proteins produces the strongest response?
- How broad is the immune response – does it work against all types of HIV?
- How long do the responses last?
The first large trial of an HIV vaccine reported results in 2003. The vaccine used in that trial, AIDSVAX, was designed to stimulate the production of antibodies against a region of an HIV surface protein, gp120. The trial found that AIDSVAX was no more effective than a placebo, or dummy vaccine, in preventing HIV infection.
Another vaccine approach was tested in a large trial called STEP. This trial tested a vaccine designed to encourage cellular immune responses. The vaccine used an adenovirus (Ad5) which causes common cold symptoms to deliver HIV proteins safely. The trial was halted in 2007 after an interim analysis showed that the vaccine had not reduced the risk of infection. Further analysis found that people with the highest levels of antibodies to the adenovirus used in the vaccine had the highest risk of becoming infected with HIV after receiving the vaccine, at least during the early phase of the trial. This study showed that care needs to be taken in the choice of virus, or vector, used to deliver HIV proteins in a vaccine.
Another study of a vaccine using the Ad5 vector but containing subtype B HIV proteins showed no effectiveness and an increase in the risk of HIV infection for vaccinated men after the study was unblinded. It is still unclear why the risk of infection increased in these participants.
An alternative approach designed to stimulate both cellular immunity and antibody production was tested in the RV144 trial. This study used two vaccines in what is called a ‘prime-boost’ approach. A vaccine called ALVAC-HIV was used to ‘prime’ the cellular immune system, using three sequences of HIV proteins. The AIDSVAX vaccine was used to boost the immune response later. ‘Prime-boost’ vaccines are designed to produce strong and long-lasting immune responses.
The RV144 trial showed that the prime-boost combination reduced the risk of infection by 31%. Many researchers were surprised by this result, as AIDSVAX had not protected against infection when used alone. Another surprising result was that the vaccine did not produce strong CD8 T-cell responses in a majority of participants and did not result in reduced viral load in people who became infected despite vaccination. The vaccine did produce strong antibody responses to a region of the HIV surface protein.
Further analysis showed that specific antibody responses which also encouraged innate-cell immune responses (antibody-dependent cell-mediated cytotoxicity, ADCC) were strongly associated with a reduced risk of infection in vaccine recipients. This finding encouraged researchers to test further prime-boost vaccine strategies.
A version of the vaccine combination used in the RV144 trial adapted for the type of HIV common in southern and eastern Africa (subtype C) was tested in the HVTN 100 study. The study found the vaccine produced very strong antibody responses of the type associated with protection against infection in the RV144 trial.
The vaccine was then tested in a much larger trial in southern Africa. The Uhambo study (also known as HVTN 702) recruited 5407 people and aimed to test whether the vaccine could reduce the risk of HIV infection by at least 50%. The trial was also set up to find out whether strong immune responses to HIV would last longer with this vaccine than with the one used in the RV144 trial, where its protective effect began to wane after one year.
However, the Uhambo trial was stopped early in February 2020 because an interim review found that the vaccine was ineffective. This was a major setback. Researchers hypothesised that the number and variety of different viral strains circulating in southern Africa, and which participants were exposed to, overwhelmed any weak immune response the vaccine might have stimulated. In contrast, RV144 had been conducted in Thailand in a lower-risk population with less circulating viral diversity.
A different prime-boost vaccine approach was tested in another large study in southern Africa. This vaccine approach had produced strong immune responses in animal studies and in preliminary human studies. The Imbokodo study (also known as HVTN 705) used a ‘prime’ vaccine consisting of an adenovirus vector that delivered a ‘mosaic’ of HIV envelope and internal proteins from four HIV subtypes designed to produce responses against a wide range of HIV subtypes. The booster vaccine used had been shown to stimulate the production of antibodies against the HIV envelope protein gp140.
Imbokodo recruited 2637 women in five southern African countries. It was a second major disappointment when Imbokodo also closed early in August 2021. Whereas in Uhambo there were equal numbers of infections in vaccine and placebo recipients, in Imbokodo 25% fewer women receiving vaccine were infected than women receiving placebo. However, this difference was so small that it was not statistically significant, in other words could have been due to chance alone.
Another study, the Mosaico trial (also known as HVTN 706) uses, as its name indicates, the same mosaic vaccine approach as Imbokodo. Mosaico began recruiting participants in North America, Latin America and Europe in 2019. This trial uses a prime and booster designed to produce responses to subtype B HIV which predominates in Europe, North America and Latin America. Recruitment of gay and bisexual men and transgender people began in those regions in 2019, and the trial is ongoing, with results expected in March 2024.
Will a vaccine protect everyone?
It is widely expected that the first generation of HIV vaccines will only be partially effective. Some people will have weaker immune responses after vaccination or will miss doses of the vaccine and fail to achieve protection. The RV144 vaccine reduced the risk of infection by only 31% but trials of newer vaccines are looking for reductions in the risk of infection of at least 50%, and preferably 65% or more, to move forward. A vaccine which only halved the risk of infection may nevertheless be highly cost-effective in regions of the world where rates of infection are high and the cost of providing treatment will continue to grow if the rate of infection cannot be reduced.
When will an HIV vaccine be available?
Scientists have swung between optimism and pessimism about the chances of developing an effective vaccine over the past 30 years. Scientists were feeling more optimistic after the results of the phase I and II studies that fed into Uhambo and Imbokodo, but we are now in a period, if not of pessimism, of retrenchment.
Even if Mosaico produces positive results after 2024, it will take several years for the results to be fully analysed and submitted for regulatory approval – and they will only apply to the subtype B epidemic that is predominant in Europe and the Americas. Vaccine manufacturing will need to be scaled up and money will need to be pledged by donors to pay for HIV vaccination campaigns in lower-income countries. Further studies will be needed to find out if the vaccine effective in this one clinical trial show similar efficacy in other populations.
If Mosaico fails, it will not mean that HIV vaccine research will stop. However, it may be the last vaccine candidate that elicits non-neutralising antibodies. These don’t directly block viral replication but recruit an immune response that does.
The next generation of vaccine candidates will need to elicit broadly neutralising antibodies (bNAbs), which do directly block viral replication, or stimulate a very broad and potent T-cell response. Novel vaccines are being researched, though they are only in phase I (safety) or preclinical studies so far. You can read more about them on another page.