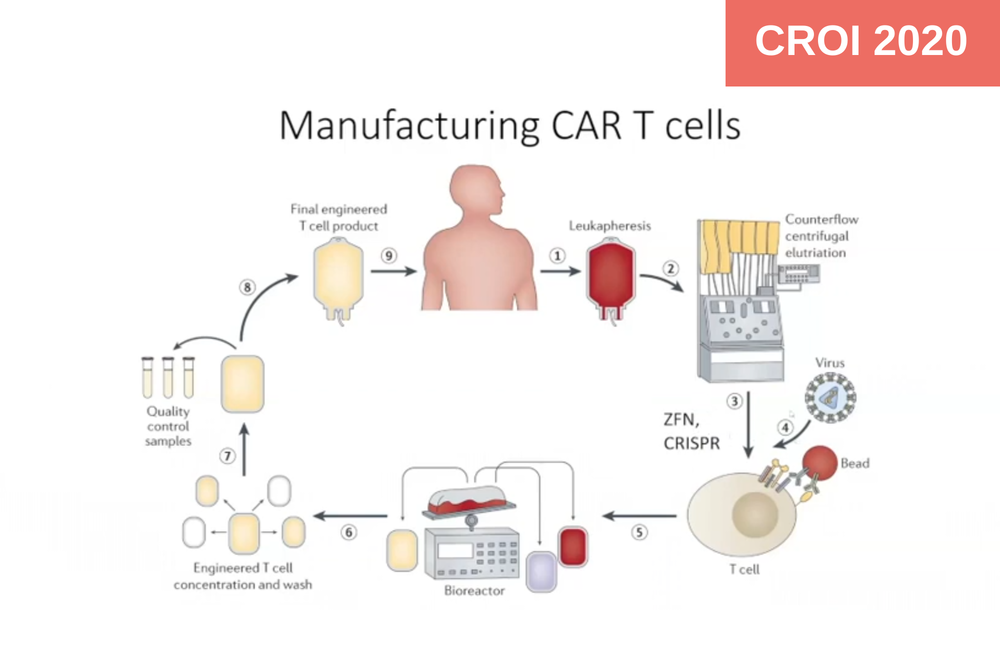
Two different approaches towards achieving a cure for HIV produced tantalising new data at the recent Conference on Retroviruses and Opportunistic Infections (CROI 2020). This annual conference was due to take place in Boston from 8 to 11 March but in-person attendance was cancelled two days before the conference was due to open and was replaced by online presentations. Even more impressively, the Community Cure Workshop, which has been held the day before CROI for several years, was cancelled at one day’s notice but still happened via live streaming.
The symposium heard opening talks by two researchers engaged in different aspects of cure research, who provided background and results data that expanded on abstracts they later presented at the conference.
Veteran HIV researcher Professor Dan Barouch of Harvard Medical School presented results from studies in monkeys that combined three different types of therapy:
- the immune stimulant drug vesatolimod to flush as many virus-infected cells as possible out of hiding;
- broadly neutralising antibodies (bNAbs) to target and kill as many as possible of them; and
- a therapeutic vaccine to create longer-term immune surveillance to mop up any remaining replication-competent virus produced by the residual infected cells.
Barouch is a lead investigator in I4C – Immunotherapy for a Cure – which is one of the Martin Delaney cure collaboratories, six research consortia funded by the US National Institute of Allergies and Infectious Diseases, whose head, Dr Anthony Fauci, has been in the news recently as the lead scientist in the US drive against the COVID-19 virus. They were named after the AIDS activist and founder of Project Inform, Martin Delaney, who died in 2009.
One of the other Delaney collaboratories is BEAT-HIV, which concentrates on gene therapy – the use of techniques that physically alter the genetic core of cells, and thus the ‘jobs’ that they can do. Dr Jim Riley of the University of Pennsylvania introduced results from this exciting area: the engineering of cells to make them immune to HIV, to induce them to make their own virus-blocking compounds, and even introduce completely ‘redesigned’ T-cells – so-called chimeric antigen receptor (CAR) cells – that produce a more efficient immune reaction to HIV than that found in nature.
Combining antibodies, immune stimulants and vaccines
Barouch's study combining vesatolimod, a bNAb and a therapeutic vaccine began with infecting 51 rhesus macaques with the artificial human/monkey spliced virus SHIV (a research tool not found in the wild). Nine days later they started on antiretroviral therapy (ART).
After six months on fully suppressive ART, 24 of the 51 monkeys received the first of four shots of a therapeutic vaccine containing SHIV genes within two different vectors (the shells of two viruses called ad26 and MVA, previously both used in human trials). About six months after that, 36 of the 51 monkeys – including the 24 vaccine recipients – started a course of ten doses of the immune-stimulating drug vesatolimod (previously known as GS-9620). About three months after that, 24 monkeys – including 12 of the monkeys who had received the vaccine – also started a course of five fortnightly infusions of the bNAb PGT121.
This means that 15 monkeys only received placebo treatment; 12 received vesatolimod and PGT121; 12 received vesatolimod and the ad26/MVA vaccine; and 12 received all three treatments.
About three months after the last dose of vesatolimod, and the last antibody infusion in those monkeys that had it (a year and eight months after they were first infected), ART was stopped and the monkeys’ viral loads were followed for the next 4.5 months.
The viral loads of the 15 animals given placebos all rebounded (reappeared). The peak viral load occurred two weeks after ART was stopped and ranged from thousands to millions of copies/ml. By 12 weeks after ART was withdrawn, these monkey’s viral loads had declined to a steady state of about 2000 copies/ml on average, with three over 10,000 and one over 100,000.
In contrast the viral loads in the 12 monkeys given the ad26/MVA vaccine plus vesatolimod also all rebounded, but to a low average level (about 200 copies/ml, with none above 2000). However, three of the monkeys re-suppressed their virus and were found to have undetectable viral loads four, six and eight weeks respectively after ART was stopped, and remained that way at 12 weeks.
In the 12 monkeys given vesatolimod plus the PGT121 bNAb, the viral load in four of the monkeys did not rebound at all, or had not done by 12 weeks. The peak viral loads in the other eight averaged about 2000, and was somewhat delayed, with the peak at between three and six weeks after ART withdrawal. However, the steady-state viral load was not much reduced from that in the animals on placebo.
Results were only available by the time of the conference for ten of the 12 monkeys given all three therapies. In four of these, the viral load did not rebound at all, and two of the others re-suppressed their viral load by week 6 and week 12, so by that time six of the ten monkeys had undetectable viral loads. In the four that did rebound and did not re-suppress, the average viral load at week 12 was about 1000.
A separate study (Prague) pooled the results from this and several other money studies of the same three therapies, such as this study reported in 2018, and looked at the contribution of the three components towards viral suppression.
When used alone, the vaccine suppressed reactivation of latent virus to 25% of what it was on placebo; it boosted the avidity of antiviral immune responses 17-fold, and 210-fold when combined with vesatolimod. The bNAb boosted immune response avidity eightfold, but combining it with vesatolimod displayed no further synergy (they did not boost immune responses more than they did separately).
If these results were replicated in humans – which is a big 'if', as other animal studies have shown – vesatolimod alone, vesatolimod plus vaccine, and vesatolimod plus antibody are expected to lead to control of viral rebound in about 5%, 55% and 90% of patients. However, this would often be after a high peak viral load and results are expected to vary widely between individuals.
At the main conference, Dr Devi SenGupta of Gilead Sciences presented the first results of using vesatolimod alone in humans, in an initial safety and viral-rebound study.
"Six of ten monkeys given all three therapies had undetectable viral loads."
In this study 17 people with HIV on ART were given ten fortnightly doses of vesatolimod and another eight people were given a placebo. They all had to be 'partial HIV controllers', meaning that their average viral load before they started ART had to be no higher than 5000 copies/ml. After the course of vesatolimod, ART was then stopped, to be restarted after six weeks, or later if the viral load was still suppressed consistently below 200. The average age of participants was 45, four out of the 25 were women, they had been on ART for an average of three years, and their average viral load before starting ART was 1585 copies/ml.
Vesatolimod, as an immune stimulant, caused some ‘fluey’ side effects: chills and headaches, along with swollen lymph nodes. On average, its effects were rather slight, with a small but statistically significant increase in the time to a viral load rebound of over 200 from four weeks on placebo to five weeks on the drug.
However, as often seen in these cure trials, a subset of participants responded more strongly, with four having no viral load rebound to over 50 within six weeks, and one not rebounding till week 15. This person took 31 weeks to reach any viral load above 200 and in fact stayed off ART for the remaining six months of the study, with a viral load varying between 164 and 215.
Gene therapy can make cells immune to HIV, or hyper-reactive to it
Jim Riley outlined the latest research in the area of genetic engineering at the Cure Workshop.
The two named people we now know to have been cured of HIV – Timothy Ray Brown and Adam Castillejo – owe their cures to an aspect of genetic manipulation. Both had bone marrow transplants of cells that lacked a crucial receptor, CCR5, that is generally needed for the virus to enter them.
The donors naturally lacked the CCR5 receptor, whereas gene therapy uses techniques to artificially create such cells. It uses molecular tools such as Zinc Finger Nucleases (ZFNs) or CRISPR-Cas9, gene-editing technologies that can physically remove or insert gene sequences out of or into cells. These gene-editing techniques only started to be used as anything more than a genetic research tool about 15 years ago, and less than ten years ago in HIV. The first experiments to edit the CCR5 gene out of people with HIV were reported by aidsmap.com in 2011.
Gene editing as a technique is far more advanced in cancer therapy than in HIV. Riley said that a search of the www.clinicaltrials.gov database showed that there were only seven clinical trials underway of gene-therapy for HIV infection, compared to 711 studies in cancer.
This is partly because HIV gene-editing techniques have to be especially safe as we already have therapies to control HIV, but scientists can take more risks to improve the survival rate from otherwise lethal cancers. As Riley put it, HIV is a “much taller fruit to reach” because it is already a manageable disease. When he is asked whether a treatment that could cost $100,000 a time would ever be cost effective, his answer is that the cancer therapy research would lead to therapies that worked for HIV and also that, as they became more common they would become cheaper.
“We may be at the era of developing mainframe computers to cure HIV right now," he said. "In 20-30 years’ time we may have laptops.”
These seven HIV gene therapy studies are aiming to do remarkable things, however.
One study uses a molecular intervention called CAL-1 that not only inactivates the CCR5 receptor from bone marrow and white blood cells, but also induces the cells to produce a fusion inhibitor that actively repels HIV infection.
"The issue is not just how many altered T-cells you can make, but also how many you can engraft."
Two techniques create HIV-resistant CD34+ cells. These are the so-called hematopoietic stem cells that normally only live deep in the bone marrow or in the umbilical cords of foetuses and diversify into the whole repertoire of immune-system cells. The aim is to see if by altering these we could develop a whole HIV-resistant T-cell system.
Another programme we have already reported on: the development of a vaccine containing the genetic instructions for cells to make broadly neutralising antibodies against HIV.
Riley is involved in two research programmes. One continues the programme of generating CCR5-resistant CD4 cells and ‘engrafting’ them into people’s immune systems.
The other involves making so called Chimeric Antigen Receptor T-cells – CAR cells. This technology physically alters T-cells that are not responsive to HIV – most aren’t, even in people with HIV – by inducing them to display cellular receptors that recognise HIV. These cells then mount anti-HIV immune responses that are different from those seen in nature, and which HIV has developed no immunity to. It is hoped that such cells could form a population of cells ‘hyper-reactive’ to HIV that could quench infections before the virus starts overwhelming the natural immune response.
Both these techniques are done by extracting T-cells from the person with HIV, altering them genetically with the tools mentioned above such as ZFNs and CRISPR-Cas9, and reinfusing the T-cells into the same patients.
The difficulty with both of these approaches is the number of T-cells that can be made. “In the first experiments with autologous engineered T-cells, we made about 10 billion T-cells lacking the CCR5 receptor," said Riley. “But there are about 500 billion T-cells in your body. So that’s about 2%. This is very different from the situation with someone like Timothy Ray Brown whose T-cells were 100% CCR5-negative, because he had a bone marrow transplant that replaced all of them.”
The issue is not just how many altered T-cells you can make, but also how many you can engraft. In other words, what proportion, out of all the T-cells in the body, are the altered ones, and whether they are able to persist and even proliferate.
Nonetheless, even in one of the earliest experiments that involved deleting the CCR5 receptor from T-cells and then re-infusing them, a pattern was seen whereby the recipients tended to have slower rebounds of their viral load when ART was stopped. More surprisingly, viral loads in all six patients in that study peaked at week 5-7 of the treatment interruption and then started to drop for the rest of the 12 weeks in which ART was withdrawn. One participant became undetectable again.
When this patient’s genes were analysed, they were found to be CCR-5 heterozygotic; that is, one of their parents had lacked the CCR5 gene, so they only had half the usual complement of CCR5 genes and fewer T-cell receptors.
In the most recent study of this technique, the University of Pennsylvania team reinfused CCR5-negative autologous T-cells back into 13 volunteers. In ten of these volunteers, they also gave them doses of the immune-suppressant drug cyclophosphamide before they infused the T-cells. Six volunteers were heterozygous and only had one copy of the CCR5 gene (if you have both copies, the term is homozygous). They also varied the dose of cyclophosphamide.
The overall results were disappointing, Riley commented. Although the delay in time to peak viral load on rebound was longer than in a control group (p=0.03), it was actually only delayed by one to two weeks. The time to 50% of the cohort rebounding was three weeks in the control group, four weeks in homozygous participants, and five weeks in heterozygous participants.
However, in three participants, viral load declined again during the time off ART, with one volunteer becoming briefly undetectable, one almost so before developing a long period with a viral load of about 1000 copies/ml, and another whose viral loads stayed at about 1000 for a prolonged period. Their viral loads 'bounced around' unpredictably, Riley said, suggesting a dynamic process happening in the engraftment of the cells. The three patients with the viral loads that bounced around had the highest proportion of altered T-cells – about 1.44% of their total complement.
The next stage for Riley’s team is to do a study combining these CCR5-depleted T-cells with CAR T-cells. As explained above, these are T-cells that have been genetically engineered to display receptor molecules on their surfaces that are hypersensitive to HIV and display different patterns of immune response than the HIV-1 specific T-cells that develop naturally.
In experiments on mice, animals given one type of CAR T-cell developed viral loads upon treatment interruption that were 2 logs (100-fold) lower than in control-group mice.
And in a study presented at CROI (Rust), four monkeys given CAR T-cells experienced delays in their viral rebound following ART withdrawal, with one still undetectable more than seven weeks after ART was withdrawn.
In the human trials, one cohort will have their ART stopped as soon as the combination of CCR5-depleted and CAR T-cells are infused; in the other, ART interruption will be delayed by eight weeks. The first patient to take part in the study had their genetically altered T-cells infused on 3 March, four days before the cure symposium.
A programme and links to the slides and video presentations at the Cure Community Workshop 2020 is at www.treatmentactiongroup.org/webinar/pre-croi-community-hiv-cure-research-workshop-2020/.
Watch Dan Barouch's video on YouTube.
Watch Jim C Riley's video on YouTube.
Abstracts presented at the main conference mentioned in this piece are as follows:
Barouch D et al. Combined active and passive immunization in SHIV-infected rhesus monkeys. Conference on Retroviruses and Opportunistic Infections, abstract 78, March 2020.
View the abstract on the conference website.
Watch the webcast on the conference website.
Prague M et al. Viral rebound kinetics following single and combination immunotherapy for HIV/SIV. Conference on Retroviruses and Opportunistic Infections, abstract 320, March 2020.
View the abstract on the conference website.
View the poster on the conference website.
SenGupta D et al. Safety and analytic treatment interruption outcomes of vesatolimod in HIV controllers. Conference on Retroviruses and Opportunistic Infections, abstract 40, March 2020.
View the abstract on the conference website.
Watch the webcast on the conference website.
Update: Following the conference presentation, this research was published in a peer-reviewed journal:
SenGupta D et al. The TLR7 agonist vesatolimod induced a modest delay in viral rebound in HIV controllers after cessation of antiretroviral therapy. Science Translational Medicine, 13, June 2021. DOI: 10.1126/scitranslmed.abg3071
Rust B et al. Chimeric antigen receptor T-cells control SHIV replication in post-ATI macaques. Conference on Retroviruses and Opportunistic Infections, abstract 76, March 2020.